1 BCDP, Program Resources Inc., NCI-Frederick Cancer
Research Facility, Frederick, MD 21701, USA
2 Laboratory of Viral Carcinogenesis, NCI Frederick Cancer Research
Facility, Frederick MD 21701, USA
3 Howard Hughes MedicalInstitute, University of Michigan, Ann Arbor,
MI, USA
* This project has been funded at least in part with Federal Funds
from the Department of Health and Human Services
under contract number N01-CO-74102 with Program Resources, Inc.
The content of this publication does not necessarily
reflect the views or policies of the Department of Health and Human
Services, nor does mention of trade names, commercial products,
or organizations imply endorsement by the U.S. Government
A. Introduction
The techniques of molecular biology have had a dramatic effect
on the advancement of human genetics. In particular, the development
of restriction fragment length polymorphisms (RFLPs) has allowed
researchers to generate genetic markers for virtually any region
of the human genome. Most RFLPs occur when a mutation creates or
deletes a recognition site for a restriction enzyme, generating
a DNA fragment of altered size. In the simplest case this will create
two alleles. A DNA probe which hybridizes to this fragment will
detect the presence of these alleles in the DNA from different individuals.
Probes used to detected RFLPs have been derived from both cloned
genes and randomly isolated DNA segments. Thus, each RFLP is a genetically
inherited marker for a precise location on a chromosome. By analyzing
the inheritance of RFLPs in families, linkage analysis techniques
can be used to determine the order and genetic distance between
different polymorphic probes [1]. When this information is combined
with data on the physicallocation of the probes, agenetic map of
a chromosome can be constructed [2]. Genetic maps are powerful tools,
useful in the detailed molecular analysis of biological problems.
By studying the inheritance of polymorphisms in families afflicted
with a genetic disease, the approximate chromosomal location of
the gene responsible for the disease can be determined. Linkage
with RFLP markers has been detected for several human disease genes
including Huntington's disease [3], muscular dystrophy [4], and
cystic fibrosis [5-7]. Linkage is often a critical step in the eventual
isolation of the gene itself [8]. RFLPs have also provided critical
data in the analysis of specific chromosomal abnormalities in human
tumors. DNA markers can be used to distinguish between the two homologous
chromosomes in the normal cells of a patient and, when compared
with DNA from a tumor, can detect the loss of a specific chromosome
or chromosomal region. Such analysis helped to identify the region
containing the gene responsible for retinoblastoma [9] and regions
containing putative genes involved in Wilm's tumor and renal cancer
[10,11] among others. In this report we present data on several
newly detected RFLPs in biologically important genes (Table 1),
describe in detail the identification of new RFLPs in three loci,
and discuss the potential usefulness of genetic polymorphisms in
the analysis of human disease.
B. Results and Discussion
The c-raf:.1 gene is a member of the family of serine protein
kinases and is the cellular homologue of the v-raf gene. vraf was
identified as the transforming gene of the murine 3611 acute transforming
retrovirus [12]. It is believed that part of the function of the
c-raf-1 gene is to act as an intracellular signal transmission molecule
for certain hormones or growth factors [13]. The human c-raf-1 gene
has been localized to chromosome 3 at bands p24-25 [14]. Using cDNA
probes from the human c-raf-1 gene, we have searched for the presence
of RFLPs in this locus. The pTuc-8 probe detects a polymorphism
with the enzyme EcoRI, with alleles of15 and 12 kb (Fig. 1). In
an analysis of 71 unrelated Caucasians, we found a frequency of
0.87 for the 15-kb allele and 0.13 for the 12-kb allele (Table 1).
In addition, the EcoRI poly
Table 1. Newly described RFLPs
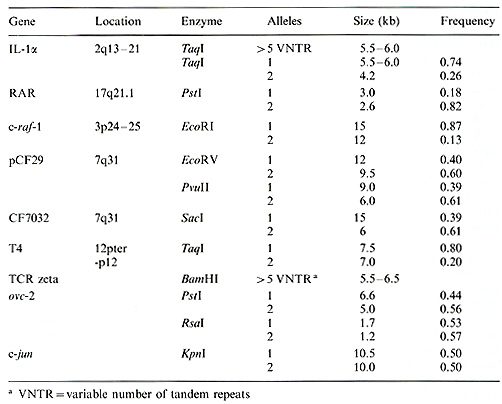
Fig.1. EcoRI RFLP in the c-raf-l gene. DNA from nine
individuals was hybridized with the pTUC-8 cDNA probe.
Size of the alleles in kilobases (kb)
morphism was shown to segregate properly in three large three-generation
pedigrees (data not shown). Specific deletions in the short arm
of chromosome 3 have been described in patients with small-cell
lung cancer (SCLC) and renal cell carcinoma [15,16]. U sing RFLPs
within the c-raf-110cus, we have examined DNA from a total of 83
human lung carcinomas (Sidthansen et al., unpublished). In an analysis
of ten paired (normal versus tumor) SCLC DNA samples, five informative
cases showed loss of heterozygosity at this locus. A BglI polymorphism
which shows 50% heterozygosity in a normal population was used to
analyze 73 unpaired lung carcinoma DNAs. Fifteen of 31 non-SCLC
samples showed heterozygosity; however, none of the 42 SCLC samples
were heterozygous. This striking apparent loss of heterozygosity
at the c-raf-1 locus in SCLC provides evidence that the c-raf-1
locus is deleted in smallcell lung carcinoma. Recently, Siezinger
et al. [17] used RFLPs in the c-raf-l gene to detect linkage between
this gene and the gene responsible for Von Hippel-Lindau syndrome,
an inherited cancer syndrome. The polymorphism described here can
be used to further study families with this disease in order to
pinpoint the location of the gene. We used probes from the interleukin-1alpha
(IL-1alfa) gene to screen for RFLPs at this locus. IL-1alfa is a
secreted protein that is involved in the stimulation of the growth
of lymphocytes. While secreted principally from macrophages, the
protein is also produced from keratinocytes and lymphocytes and
plays a role in the stimulation of lymphocytes and fibroblasts [18].
Using an IL-1alfa cDNA probe we detected a two-allele polymorphism
with the enzyme TaqI, and found that these alleles have frequencies
of 0.74 and 0.26 (Table 1 ). In addition, we found that a fragment
of this gene, detected by several different enzymes, showed a high
degree of variability between individuals. This type of variation
is characteristic of
Fig. 2. Polymorphisms in the intcrleukin-l-alpha gene.
TaqI detects a two-allele site
polymorphism as well as a high polymorphic VNTR
polymorphisms known as VNTRs (variable number of tandem repeats).
VNTRs are regions of DNA containing a group of tandem-repeated sequences
[19]. The number of repeats at a given locus is often highly variable
between different individuals, making these polymorphisms very informative
for genetic analyses. Examination of the published genomic sequence
of the genomic IL-l alfa gene [20] revealed the presence of a group
of tandem repeats in the intervening sequence between the fifth
and sixth exons (Fig. 2). The sequence of these repeats is typical
of those found in other VNTRs which have been characterized.. The
ILlalfa gene has been mapped to chromosome 2q13-21 and the polymorphisms
we have described will be useful genetic markers for this region.
In addition, these polymorphisms can be used to test whether the
IL-l alfa gene is linked to any human genetic disease for which
family pedigrees are available.
Fig. 3. Polymorphisms for probes tightly linked to the
CF gene. The positions of the chromosome-jumping clones CF 70-32,
CF 31, and CF 29 are shown in relation to the pJ3.11 probe. RFLPs
detected by these clones are displayed with sizes in kilobases.
S, SacI; M, MspI; T, TaqI; P, PvulI; E, EcoRV
Cystic fibrosis (CF) is the most common fatal genetic disease
among Caucasians; it is caused by a recessive mutation at a single
locus. The gene responsible for cystic fibrosis has been linked
to polymorphic markers that map to chromosome 7q31 [21]. Recently,
the location of the gene has been narrowed to a 700-kb region between
the probes XV2c and pJ3.11 [22]. In order to clone and characterize
additional sequences from this region, we have used chromosome jumping
[23, 24] to isolate sequences surrounding the pJ3.11 locus. Briefly,
chromosome jumping involves the circularization of large DNA fragments
and the cloning of the ends of such fragments. By generating a library
of chromosome-jumping clones and screening with a probe from the
pJ3.11 locus, we have been able to isolate sequences spanning 300-400
kb surrounding pJ3.11 (Fig. 3). Using probes from this region we
have searched for RFLPs and have discovered three new polymorphisms
to date (Table 1). Two of these RFLPs are detected with the CF29
probe, which is located approximately 150 kb from pJ3.11, away from
the CF gene. The third RFLP is detected with CF70-32, a jumping
clone that lies closer to the CF gene (Fig. 3; unpublished data).
While all three of these RFLPs are fairly informative, in preliminary
analysis they all show a high degree of linkage disequilibrium with
the MspI RFLP detected by pJ3.11. In other words, all four of these
RFLPS reveal very little new information and increase the heterozygosity
only from 53% to 58%.
C. Conclusion
Further progress in the molecular analysis of human genetic disease
will rely on the development of increasingly detailed chromosome
maps. At present, most of the maps of human chromosomes were constructed
principally with anonymous DNA markers, not known to be part of
genes. We have begun to characterize RFLPs in biologically important
genes, many of which have been mapped to specific chromosomallocations
by in situ hybridization. The list of genes in which we have detected
RFLPs includes the proto-oncogenes and growth factors craf-1, c-jun,
c-ovc-2, and IL-l alfa, and cell surface molecules and receptors
such as the retinoic acid receptor, the T -cell receptor zeta gene,
and the T 4 gene, receptor for HIV. In addition to providing markers
for specific chromosomallocations, the RFLPs in these genes will
allow them to be tested as candidate genes for human genetic diseases.
References
1. Botstein D, White R, Skolnick M, Davis RW (1980) Construction
ofa genetic linkage map in man using restriction fragment length
polymorphisms. Am J H um Genet 32:314-331
2. White R, Leppert M, Bishop DT, Barker D, Berkowitz J, Brown
C, Callahan P , Holm T, Jerominski L (1985) Construction of linkage
maps with DNA markers for human chromosomes. Nature 313:101-105
3. Gusella JV, Wexler, NS et al. (1983) A polymorphic DNA marker
genetically linked to Huntington's disease. Nature 306:234
4. Davies KE, Pearson PL et al. (1983) Linkage analysis of two
cloned DNA sequences flanking the Duchenne muscular dystrophy locus
on the short arm of the human X chromosome. Nucleic Acids Res 11:2303-2312
5. Tsui L-C, Buchwald M, Barker D, Braman JC, Knowlton R et al.
(1985) Cystic fibrosis locus defined by a genetically linked polymorphic
DNA marker. Science 230: 1054-1057
6. Wainwright BJ, Scambler PJ, Schmidtke J, Watson EA, Law H-
Y, Farrall M, Cooke HJ, Eiberg H, Williamson R (1985) Localization
of cystic fibrosis locus to human chromosome 7cen-q22. Nature 318:
384-386
7. White R, Woodward S, Leppert M, O'Connell P, Hoff M, Herbst
J, Lalouel J-M, Dean M, Vande Woude G (1985) A closely linked genetic
marker for cystic fibrosis. Nature 318:382-384
8. Monaco AP, Neve RL, Colletti-Feener C, Bertelson CJ, Kurnit
DM, Kunkel LM (1986) Isolation of candidate cDNA's for portions
of the Duchenne muscular dystrophy gene. Nature 323:646-650
9. Cavenee WK, Dryja TP, Phillips RA, Benedict WF Godbout R, Gallie
BL, . Murphree AL, Strong LC, White RL (1983) Expression of recessive
alleles by chromosomal mechanisms in retinoblastoma. Nature 305:
779- 784
10. Koufos A, Hansen MF, Copeland NG, Jenkins NA, Lampkin BC, Cavenee
WK (1986) Loss of heterozygosity in three embryonal tumors suggests
a common pathogenetic mechanism. Nature 316: 330- 334
11. Zbar B, Brauch H, Talmadge C, Linehan M (1987) Loss of alleles
of loci on the short arm of chromosome 3 in renal cell carcinoma.
Nature 327: 721
12. Rapp UR, Goldsborough MD et al. (1983) Structure and biological
activity of v-raf, a unique oncogene transduced by a retrovirus.
Proc Natl Acad Sci USA 80:4218
13. Rapp UR, Storm SM, Cleveland JL (1987) Oncogenes: Clinical
Relevance. Hematology 31 :450-459
14. Bonner T, O'Brien S1, Nash WG, Rapp UR (1984) The human homologs
of the raf (mil) oncogene are located on human chromosomes 3 and
4. Science 223: 71- 74
15. Whang-Peng 1, Kao-Shan CS, Lee EC (1982) Specific chromosome
defect associated with human small-ccll lung cancer: deletion 3p(14-23).
Science 215:181-182
16. Kok K, Osinga 1, Carritt B, Davis MB, van der Hout AH, van
der Veen AY, Landsvater RM et al. (1987) Deletion ofa DNA sequence
at the chromosomal region 3p21 in all major types of lung canccr.
Nature 330: 578-581
17. Seizinger BR, Roleau GA et al. (1988) Yon Hippel-Lindau disease
maps to the region of chromosome 3 associated with renal cell carcinoma.
Nature 332: 268 -269
18. Oppenheim 11, Kovacs E, Matsushima K, Durum SK ( 1986) There
is more than one interleukin 1. Immunol Today 2: 45- 56
19. Nakamura Y, Leppert M, O'Connell P, Wolfe R, Holm T, Culver
M, Martin C, Fujimoto E, Hoff M, Kumlin E, White R (1987) Variable
number of tandem repeat (YNTR) markers for human gene mapping. Science
235: 1616-1622
20. Furutani Y, Notake M, Fukui T, Ohue M, Normura H, Yamada M,
Nakamura S (1986) Complete nucleotide sequence of the gene for human
interleukin 1 alpha. Nucl Acids Res 14: 3167 -3179
21. Dean M (1988) Molecular and genetic analysis of cystic fibrosis.
Genomics 3:93-99
22. Drumm ML, Smith CL, Dean M (1988) Physical mapping of the cystic
fibrosis region by pulsed-field gel electrophoresis. Genomics 2:
346-354
23. Collins FS, Weissman SM (1984) Directional cloning of DNA fragments
at a large distance from an initial probe: A circularization method.
Proc Natl Acad Sci USA 81:6812-6816
24. Collins FS, Drumm ML, Cole 1L, Lockwood WK, Vande Woude FG,
Iannuzzi M C ( 1987) Construction of a general human chromosome
jumping library, with application to cystic fibrosis. Science 235:
1046-1049
|