Institute of Cancer Research and Department of Human
Genetics and Development, College of Physicians and Surgeons,
Columbia University, New York, N. Y. 10032 U. S. A.
RNA Tumor Viruses and Human Leukemias Considerable information
is available concerning the etiological role of RNA tumor viruses
in leukemias, lymphomas, sarcomas, and breast cancer in a variety
of animals, e.g., chicken (1), mice (2), cats (3), etc. In animals,
causative proof has been achieved by inoculation experiments that
satisfy Koch\'s postulates. In man, such experiments obviously cannot
be undertaken, and other more indirect evidence has to be assembled
to clarify the etiology of human malignancies with its implications
for prophylaxis and therapy.
The data reported here on the presence of viral-like particles in
human leukemias are based on the technique of molecular hybridization
of nucleic acids (4-10) and of the simultaneous detection of high
molecular weight RNA associated with a reverse transcriptase at
physical densities characteristic of oncogenic RNA viruses (11-17).
The general biochemical and physical properties of the RNA tumor
viruses in clude a characteristic density of 1.16 g/cm³. Inside
an outer shell they contain nucleoids with a density of 1.23 g/cm³
.As genetic material they possess a high molecular weight, with
60 to 70S-sedimenting RNA, which can dissociate into 35S subunits.
The RNA is associated with an RNA-dependent DNA polymerase (reverse
transcriptase) (18, 19). With this enzyme, a radioactively labeled
DNA can be synthesized that is complementary to the tumor virus
RNA (5). Several considerations (5,7) led us to the assumption that
the RNAs of different mammalian leukemia viruses might be related
to each other with regard to their base sequence. To test this assumption,
radioactive DNA , which had been synthesized on RNA of a leukemia
virus of a mouse (RL V), was hybridized to RNA isolated from leukocytes
of a leukemic rat (7). After a positive outcome of this comparison
involving two closely related animal hosts, a similar experiment
was undertaken by hybridizing RL V-³ H-DNA to RNA isolated from
the cytoplasm of human leukemic cells (7). In Fig. 1 the density
profiles are shown of hybridizations with RNAs from four human leukemias.
The ³ H-DNA mainly bands in the DNA density region of the gradient
(p = 1.45 g/cm³ ), but some of the radioactive DNA can be seen banding
in the RNA density region of the gradient (p = 1.65 g/cm³ ) complexed
to the denser RNA. Homologies to mouse leukemia virus RNA were found,
whether the human leukemic RNA was derived from lymphoblastic or
myelogenous, acute, or chronic leukemias.
Fig. 1: Cs2S04
density profiles of hybridization reactions between ³H-RLV-DNA and
cytoplasmic RNA isolated from human leukemic white blood cells. ³
H-RL V -DNA and cytoplasmic RNA were prepared as described (7). About
350 µg RNA was hybridized to 2000 cpm of purified, heat denatured
³H-RLV-DNA (10,000 cpm/pmol) for 18 hr at 37 °c in the presence of
0.4 M NaCI and 50% formamide (total vol 60 µl). After incubation,
the reaction mixture was added to 10 ml half-saturated Cs2S04 (p =
1.52 g/cm³) in 5 mM EDTA and centrifuged at 44,000 rpm in a 50 Ti
rotor (Spinco) for 60 hr at 15 °C. Fractions of 0.4 ml were collected
from below and assayed for TCA-precipitable activity. The cytoplasmic
RNA was derived from A) AML (F), B) CML (A), C) ALL (G), D) CLL (S).
Positive outcomes were observed in 28 out of 31 leukemic patients,
i. e., in more than 90% the RNA isolated from leukemic leukocytes
showed homology to the RNA of a mouse leukemia virus. In contrast,
more than 50 normal control tissues, including normal leukocytes,
did not show this kind of response.
The position of the RNA-DNA-hybrids in the CS2
SO4 density gradients indicates that the human
leukemia-specific RNA determines the density of the hybrids and therefore
is considerably larger than the 5-8S large radioactive DNA. It was
logical to assume that this large RNA is of the 60- 70S type and possibly
associated with a reverse transcriptase in a complete viral particle.
The resolution of these and related questions was made feasible by
the apllication of a technique for the simultaneous detection of a
high molecular weight RNA associated with a reverse transcriptase
(11, 12). The basis for the simultaneous detection of 60- 70S RNA
and reverse transcriptase stemmed from the observations (20-22) that
the initial DNA product is found complexed to its 70S-RNA template.
If early DNA product is found on sedimentation analysis to travel
as if it were a 70S molecule, and if supplementary evidence is provided
that its apparent size is due to its being complexed to a 70S-RNA
molecule, evidence is provided for the presence of reverse transcriptase
that uses a 70S-RNA template. Figure 2 shows representative results
of experiments along these lines with leukocytes of leukemic patients
(13,16). After an endogenous reverse transcriptase reaction and deproteinization
of the nucleic acids a portion of the ³H-DNA sediments in discrete
70S and 35S peaks in glycerol-sedimentation-gradients. After RN ase
digestion, the entire radioactive DNA is found in the low molecular
weigh t region of the gradient, as exemplified in Fig. 2B. This proves
that the ³H-DNA was complexed to a high molecular weight RNA. In Fig.
2, experiments with cell material from patients with ALL (A) and CML
(B) are shown. In total, more than 50 leukemic cell samples gave the
specific DNA-synthesis resulting in ³H-DNA com plexed to a 70S RNA
whereas only one out of 27 normal white blood cell samples yielded
evidence for this type of activity. In this case, the available white
blood cells did not permit further characterization by hybridization
and thus an unambig uous statement as to the nature of this positive
reaction cannot be made. After extensive alkali digestion to destroy
all RNA present, the ³H-DNA was recovered and annealed to viral-enriched
RNA of the same leukemic cells that had been used for the specific
DNA synthesis. From Fig. 3A, it is clear that about 20 % of the ³H-DNA
is shifted from the DNA region to the hybrid and RNA regions of the
gradient due to formation of RNA-DNA hybrid structures. when the same
human ³H-DNA is annealed with an equivalent amount of AMV-70S-RNA,
no evidence of hybrid formation is seen (3B). This back hybridization
completes the operational definition of a reverse transcriptase and
shows that human leukemias contain 70S RNA-associated reverse transcriptase.
These data are in agreement with the finding by other groups of reverse
transcriptase in human leukemic cells (23, 24 ).
Fig. 2: Simultaneous detection of 60- 70S RNA and reverse
transcriptase in human leukemic white blood cells. 0.5-2 9 of white
blood cells were disrupted in TNE buffer (0.01 M Tris-HCI, pH 7.4,
0.15 M NaCI, 5 mM EDTA) with a Dounce homogenizer. Nuclei were removed
by centrifugation of the cell homogenate at 4000 X g for 10 min at
0 °C, and tIhe supernatant was centrifuged at 10,000 X g for 10 min
at 0 °C. The resulting postmitochondrial supernatant fluid was then
layered on a 20-ml column of 20% glycerol in TNE and spun at 100,000
X g for 1 hr at 1 °C in a Spinco SW-27 rotor. The resulting pellet
was resuspended in TNE buffer (2 ml per 1 g of cells). Insoluble debris
was removed from thhis suspension by cenhtrifugation at 4000 X g for
10 min. For further purification and density determination, the suspension
was then layered on several preformed 20-60% sucrose TNE gradients
and spun for 3 hr at 100,000 X g and 4 °C. Seven to 10 fractions were
collected from each gradien1t. Each fraction was diluted in TNM buffer
(0.01 M Tris-HCI, pH 7.4,0.15 M NaCI, 2 mM MgCI2)\' pelleted (100,000
X g, 1 hr, 4 °C, Spinco SW-27 rotor), resuspended in 0.3 ml of 0.1
M NaCI --0.01 M Tris-HCI, pH 8.3, and assayed for endogenous reverse
transcriptase activity: After preincubation with 0.1 % Nonidet P-40
at 0 °C for 15 min. 3µmol MgCI2, 0.4 µmol each of dATP, dGTP, dCTP,
1 mCi³H-TTP (NEN, 50.1 mCi/mmole) an1d lOO µg/ml actinomycin D to
inhibit DNA-instructed DNA synthesis were added to give a final vol
of 0.5 ml. The reaction was incubated at 37 °C for 15 min. After adjustment
to 0.2 M NaCI to stabilize hybrid structures, the reaction was terminated
by addition of 0.5 % SDS and subsequent deproteinization by phenol-cresol
extraction. The aqueous phase was then layered on a 10-30% glycerol
in TNE gradient and centrifuged at 40,000 rpm for 3 hr at 1 °C (SW-41
rotor, Spinco). Fractions of 0.4 ml were collected from below and
assayed for TCA-precipitable radioactivity. 18 and 28S rRNA and 70S
AMVRNA served as markers. The sedimentation profiles of two representative
simultaneous detection tests with the appropriate density fractions
are shown. A) ALL (H) at density p = 1.23, B) CML (Con) at density
p = 1.16 g/cm³. RNase sensitivity was tested by incubating the reaction
mixture with 80 µg/ml ribonuclease A (Worthington) for 15 min at 37
°C prior to sedimentation analysis.
By density fractionation of the leukemic cytoplasm, it could be
shown that this 70S-RNA-DNA polymerase complex has a density of 1.16
g/cm³ (Fig. 4 ), which is characteristic of RNA tumor viruses (16).
Further, the DNA synthesized by the leukemic cell reverse transcriptase
on its own endogenous template is related in sequence to RLV RNA (13).
Note that this last result is complementary to and completes the logic
of our earlier experiments in which the DNA synthesized on RL V RNA
was used as a probe to find the virus-related information in leukemic
cells. The experiments reported here demonstrate the presence in human
leukemic cells of four parameters characteristic of RNA tumor viruses:
1) a high molecular weight RNA of the 60- 70S type, 2) a reverse transcriptase,
3) a base sequence of the RNA, which is related to that of a mouse
leukemia virus, and 4) a physical density for the 70S RNA-DNA-polymerase
complex characteristic of RNA tumor viruses. The findings are individually
suggestive of a viral agent, but do not prove a viral etiology of
human leukemia. The data, however, do encourage further efforts along
these lines.
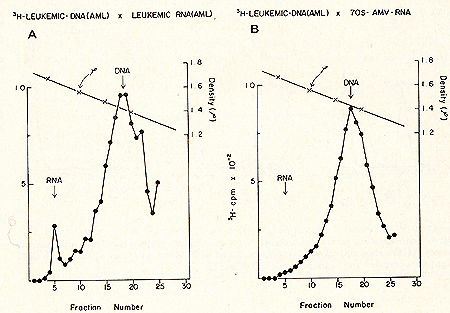
Fig. 3: CS2 SO4
equilibrium density gradient centrifugation of annealing reactions
of human leukemic (AML [TI) ³H-DNA to (A) 85 µg human leukemic (AML
[TI) RNA enriched in viral sequences by prior density fractionation
of cytoplasmic material and subsequent RNA extraction from the 1.10-1.26
g/cm³ density region, and (B) 0.5 µg highly purified 70S AMV -RNA.
A standard RNA-instructed DNA polymerase reaction was performed
as described in the legend to Fig. 2. The high molecular weight
RNA ³H-DNA complex obtained after glycerol sedimentation centrifugation
was digested with 0.4 M NaOH for 18 hr at 37 °c to remove all RNA
present. The ³H-DNA product was then annealed to the respective
RNAs in a vol of 60 µI in the presence of 50% formamide and 0.4
M NaCl. After annealing for 18 hr at 37 °C, the reaction mixture
was subjected to CS2S04 gradient centrifugation as described under
Fig. 1.
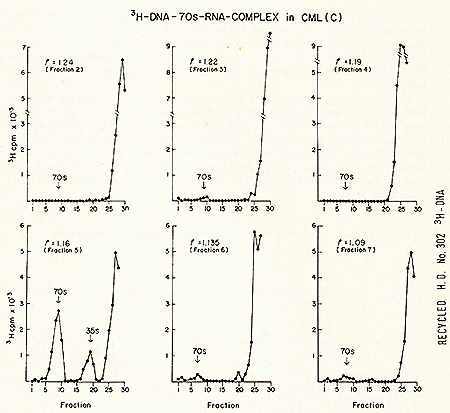
Fig.4: Sedimentation profiles of a complete set of simultaneous detection
tests after sucrose density fractionation of leukemic cytoplasm.
2 9 of white blood cells from a patient with CML (Con) were prepared
and examined as described in Fig. 2.
Acknowledgments
The excellent technical assistance of Jeanne Myers and Lee Hindin
is appreciated.
This research was supported by the National Institutes of Health,
National Cancer Institute,
Virus Cancer Program Contract 70-2049 and Research Grant CA-02332.
References
1. Rous, P.J. Am. Med. Assoc. 56: 198 (1911)
2. Gross, L.Proc. Soc. Exp. BioI. &Med. 76: 27 (1951)
3. Jarrett, W. F. H., Martin, W. B., Crighton, G.W. et al.Nature
202: 566 (1964)
4. Hall, B. D. & Spiegelman, S. Proc. Nat. Acad. Sci. USA 47: 137
(1961)
5. Axel, R., Schlom, J. & Spiegelman, S. Proc. Nat. Acad. Sci. USA
69: 535 (1972)
6. Axel, R., Schlom, J. & Spiegelman, S. Nature 235; 32 (1972)
7. Hehlmann, R., Kilfe, D. & Spiegelman, S. Proc. Nat. Acad. Sci.
USA 69: 435 (1972)
8. Kilfe, D., Hehlmann, R. & Spiegelman, S. Science 175: 182 (1972)
9. Hehlmann, R., Kilfe, D. & Spiegelman, S. Proc. Nat. Acad. Sci.
USA 69: 1727 (1972)
10. Kilfe, D., Hehlmann, R. & Spiegelman, S Proc. Nat. Acad. Sci.
USA 70; 5 (1973)
11. Schlom, J. & Spiegelman, S. Science 174: 840 (1971)
12. Gulati, S., Axel, R. & Spiegelman, S. Proc. Nat. Acad. Sci.
USA 69: 2020 (1972)
13. Baxt, W., Hehlmann, R. & Spiegelman, S. Nature New BioI. 240:
72 (1972)
14. Axel, R., Gulati, S. & Spiegelman, S. Proc. Nat. Acad. Sci.
USA 69: 3133 (1972)
15. Kilfe, D., Magrath, I. T., Ziegler, J. L. & Spiegelman, S. Proc.
Nat. Acad. Sci. USA 70: 737 (1973)
16. Hehlmann, R. & Spiegelman, S. Manuscript in preparation.
17. Duesberg, P. H.Proc. Nat. Acad. Sci. USA 60: 1511 (1968)
18. Temin, H. M. & Miziltani, S. Nature 226: 1211 (1970)
19. Baltimore, D. Nature 226: 1209 (1970)
20. Spiegelman, S., Bilrny, A., Das, M. R., Keydar, J., Schlom,
J., Travnicek, M., and Watson, K. Nature 227: 563 (1970)
21. Bishop, D. H. L., Rilprecht, R., Simpson, R. W. & Spiegelman,
S. J. Virol. 8; 730 (1971)
22. Rokutanda, M., Rokutanda, H., Green, M., Fujinaga, K., Ray,
R. V. & Gurgo, C. Nature 227: 1026 (1970)
23. Gallo, R. C., Yang, S. S., & Ting, R. C. Nature 228; 927 (1970)
24. Sarngadhavan, M. G., Sarin, P. S., Reitz, M. S. and Gallo, R.
C. Nature New Biol. 240: 67 (1972) .
|